Research
Quantum information with atoms and photons
Photons are ideal carriers of quantum information, and this information can be coherently transferred to atomic ensembles via their interaction. These atomic systems work as quantum memories which can be used for quantum information processing and long-distance quantum communications. Different degrees of freedom of single photons allow for encoding information in many dimensions, resulting in higher information carrying capacity of a single photon. We investigate how to optimize the amount of information contained in single photons and entangled photon pairs by exploring different degrees of freedom allowing for multi-level encoding, and how to transfer this information to atomic ensembles to enable high-dimensional atom-photon interfaces for quantum information.
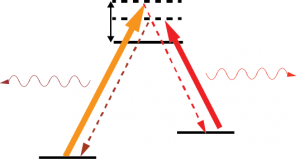
Photons can carry quantum information in different degrees of freedom. The amount of information that can be contained in photons depends on the size of the dimension of the particular degree of freedom chosen to encode information. We study the generation of photons from a spontaneous four-wave mixing FWM process in cold atomic ensembles of Cesium, which are naturally compatible with atomic quantum memories. Due to energy and momentum the conservation in the process, the generated photons are entangled in different degrees of freedom. For example, photon pairs entangled in orbital-angular momentum (OAM) have a higher capacity for information transfer, due to de high dimensionality of the OAM space. We investigate how to increase the amount of information carried by these photons, and how to create high-dimensional entanglement between atoms and multimode quantum memories. We are interested in efficient methods for the characterization of these multidimensional systems, and potential applications of these high dimensional quantum systems.
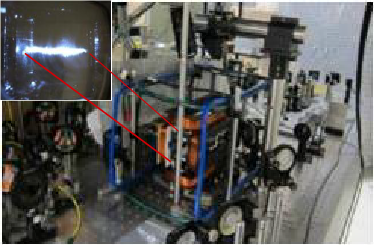
Magneto-Optical Trap of Cs atoms Setup
Quantum measurements for communications
The measurement of the state of a quantum system is limited by the intrinsic noise of such system. In particular, quantum mechanics prevents nonorthogonal states to be distinguished with total certainty. This intrinsic property of such states has undesirable consequences in optical communications, where the information encoded in such states cannot be perfectly decoded producing decoding errors and loss of information. On the other hand, nonorthogonal states are a fundamental component of quantum key distribution for secure communications. We study and realize optimized strategies for measuring nonorthogonal coherent states of light beyond the conventional limits of detection, such as the heterodyne limit, and investigate their potential for coherent and quantum communications.
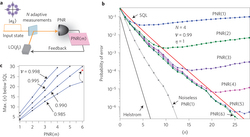
Coherent states have intrinsic quantum noise that overlaps among different states. This noise does not allow any deterministic measurement to perform discrimination with total certainty. This limits how well we can decode the informaiton encoded in nonorthogoal coherrent states, and impact the maximum amount of information that can be transmitted in communication. On the other hand, the nonorthgonality of states are central for quantum key distribution to ensure secure communications, and nonorthogonal coherent states can maximize amount of informaiton lossy channels.
We investigate different measurement strategies of nonorthogonal coherent phase states to achieve sensitivities beyond what is possible with conventional measurements performing phase-sensitive detection, such as heterodyne measurement. These nonconventional receivers have a large potential for quantum and coherent communications, and can provide insigth into the limits of information transfer under realistic conditions of real communication channels with with losses, noise and imperfect devices.
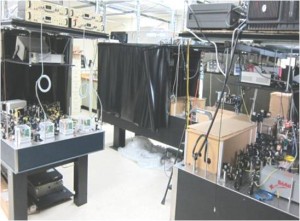
Quantum measurement Setup
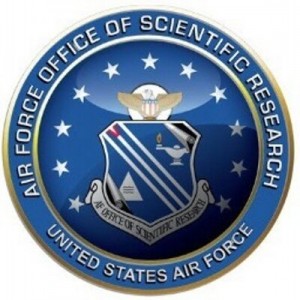