NQI Joint Algorithms Workshop
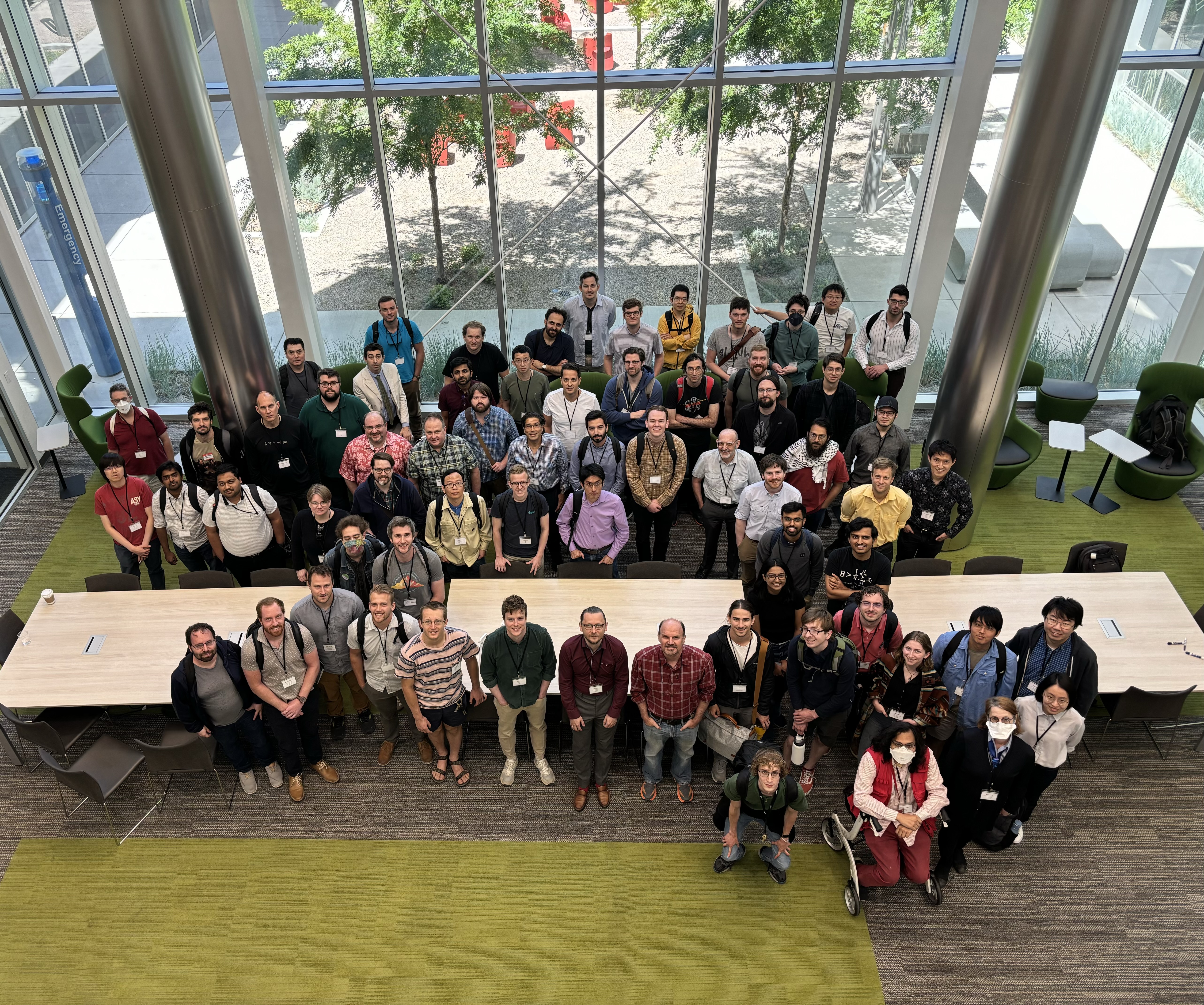
May 20, 2024 8:00 AM -
May 22, 2024 5:00 PM
UNM PAIS, and/or virtually on Zoom
The Department of Energy's National Quantum Initiative Centers will lead a workshop to accelerate the latest developments in Quantum Algorithms and applications in Quantum Information Science. This workshop is hosted by the Center for Quantum Information and Control (CQuIC) at the University of New Mexico, a National Science Foundation Focused Research Hub in Theoretical Physics. The workshop will take place on campus at the Physics and Astronomy and Interdisciplinary Science (PAÍS) building, Room 1100 and virtually over Zoom.
Organizing Committee
- Norm Tubman (SQMS)
- Nathan Wiebe (C2QA)
- Peter Love (QSA)
- Eric Chitambar (QNEXT)
- Andrew Sornborger (QSC, Chair)
This workshop will include the following invited speakers
- Jay Gambetta (IBM)
- Tom Iadecola (Iowa State, SQMS)
- Henry Lamm (Fermilab, SQMS)
- Eliot Kapit (Colorado School of Mines, SQMS)
- David Weld (UC Santa Barbara, QSC)
- Lukasz Cincio (LANL, QSC)
- Sasha Boltasseva (LANL, Purdue)
- Fred Chong (U Chicago, QNEXT)
- Bill Fefferman (U Chicago, QNEXT)
- Bryan Clark (UIUC, QNEXT)
- Kaitlin Smith (NW, QNEXT)
- Sergey Bravyi (IBM, C2QA)
- Yanzhu Chen (Virginia Tech, C2QA)
- John Preskill (Caltech, QSA)
- Peter Love (Tufts, QSA)
- Akimasa Miyake (UNM, QSA)
- Misha Lukin (Harvard, QSA)
There will be two sessions per day with two invited talks in the morning and a third in the afternoon. These will be followed by discussions.
Each session will have two or three lightning talks by Center researchers.
We will live stream and record the talks so you will be able to access them online after the event.
Registration
Registration is open to members of one of the five NQI Centers or by special invitation. For Inquiries please write to cquic@unm.edu.
Early Bird Registration will end on Friday April 5, 2024
We will have a workshop banquet on Tuesday, May 21st 2024 from 6:00 pm - 9:00 pm at Hotel Parq Central.
For in-person attendance, there will be a registration fee.
Hotel
The workshop preferred hotel is the Hotel Parq Central, 1.2 miles from UNM.
806 Central Avenue SE
Albuquerque, NM 87102
Phone: (505) 242-0040
Email: info@hotelparqcentral.com
Workshop Location
Physics and Astronomy and Interdisciplinary Sciences (PAÍS) Building
Room 1100
210 Yale Blvd NE
Albuquerque, NM 87106
Please enter by the west door through the courtyard. The other doors will be locked -- UNM Card access only
Schedule
Monday, May 20th
Introductions 8:30-9:00
Speaker: Jay Gambetta, IBM (Keynote), 9-9:40, Discussion 9:40-9:50
TBD
Speaker: David Weld (remote), QSC, UCSB, 9:50-10:30, Discussion 10:30-10:40
Title: Analog quantum simulation with ultracold atoms
Abstract: Trapped quantum gases offer an ideal context for the investigation of quantum systems driven far from equilibrium. I will discuss the general structure of such experiments from the point of view of analog quantum simulation, and present results from recent work using ultracold lithium and strontium. Time permitting, I will briefly describe a new direction in the field, towards "quantum interactive dynamics" in which measurement and feedback play a key role.
Break 10:40-11:00 (light refreshments)
Speaker: Bill Fefferman, QNEXT, U Chicago, 11:00-11:40, Discussion 11:40-11:50
Title: Quantum Pseudo-Entanglement
Abstract: Quantum pseudorandom states are efficiently preparable states that are indistinguishable from truly Haar random states to an efficient observer. First defined by Ji, Liu and Song, such states have found a wide variety of applications in areas such as quantum gravity and cryptography. A fundamental question is exactly how much entanglement is required to create such states. Haar-random states, as well as t-designs for t ≥ 2, exhibit near-maximal entanglement. Here we provide the first construction of pseudorandom states with only polylogarithmic entanglement entropy across an equipartition of the qubits, which is the minimum possible. Our construction can be based on any one-way function secure against quantum attack.
More fundamentally, our work calls into question to what extent entanglement is a “feelable” (or efficiently observable) quantity of quantum systems. Inspired by recent work of Gheorghiu and Hoban, we define a new notion which we call “pseudoentanglement”, which are ensembles of efficiently constructible quantum states which hide their entanglement entropy. We show such states exist in the strongest form possible while simultaneously being pseudorandom states and discuss applications to quantum algorithms and to the theory of quantum gravity.
This talk is based on arXiv:2211.00747 and arXiv:2311.12017.
Lunch 11:50-1:50 (buffet style taco bar)
Speaker: Eliot Kapit, SQMS, Colorado School of Mines, 2:00-2:40, Discussion 2:40-2:50
Title: What ensures approximation hardness for quantum algorithms?
Abstract: Constraint satisfaction problems are an important area of computer science. Many of these problems are in the complexity class NP which is exponentially hard for all known methods, both for worst cases and often typical. Fundamentally, the lack of any guided local minimum escape method ensures both exact and approximate classical optimization are hard, but the intuitive mechanism(s) for approximation hardness in quantum algorithms are poorly understood. For algorithms simulating Hamiltonian time evolution, we explore this question using the prototypically hard MAX-3-XORSAT problem class. We conclude that the mechanisms for quantum exact and approximation hardness are fundamentally distinct. We qualitatively identify why traditional methods such as quantum adiabatic optimization are not good approximation algorithms. We propose new spectral filtering optimization methods to escape these issues, and study them analytically and numerically. We consider random rank-3 hypergraphs including extremal planted solution instances, where the ground state satisfies a much higher fraction of constraints than truly random problems. We show that, if we define the energy to be E = Nunsat-Nsat, spectrally filtered quantum optimization will return states with (where is the ground state energy) in low-order polynomial time, where conservatively, . This is in contrast to for the hardest instances with classical searches. We thus conjecture that random hypergraph instances, including extremal instances with planted partial solutions, are efficiently approximable with high probability. We do not claim that this approximation guarantee holds for all possible hypergraphs, but at present do not know what hypergraph properties would break spectrally filtered quantum optimization. These results suggest that quantum computers are more powerful for approximate optimization than had been previously assumed.
Speaker: Jeffery Larson, QNEXT, Argonne NL, 2:50-3:05, Discussion 3:05-3:10
Title: Specialized Numerical Optimization Algorithms for QIS
Abstract: Numerical optimization problems abound across the quantum information science landscape. General-purpose numerical optimization methods often fail to identify high-quality solutions in a reasonable time. This talk highlights how newly developed specialized optimization methods can exploit problem structure to more efficiently solve some QIS problem instances. This talk provides an overview of these methods and highlights their application to problems in optimizing circuit synthesis, entanglement, and quantum Fisher information. Hopefully, these examples will spark discussions for future collaborations.
Break 3:10-3:30 (light refreshments)
Speaker: Sergey Bravyi, IBM Q, 3:30-4:10, Discussion 4:10-4:20
Title: Quantum complexity of the Kronecker coefficients
Abstract: It is commonly believed that computational problems with a group-theoretic structure may benefit from quantum algorithms. I will discuss a well-known problem in this category: computing the Kronecker coefficients. Informally, Kronecker coefficients are entries of the multiplication table for irreducible representations (irreps) of the symmetric group. Given a triple of irreps $a,b,c$, the corresponding Kronecker coefficients is defined as the multiplicity of the irrep $c$ in the tensor product of irreps $a$ and $b$. Our main result is that a given Kronecker coefficient is proportional to the rank of a projector that can be measured efficiently using a quantum computer. As a consequence, deciding positivity of Kronecker coefficients is contained in the complexity class QMA, complementing a recent NP-hardness result of Ikenmeyer, Mulmuley and Walter. As a second consequence, we obtain an efficient quantum algorithm for computing a certain restricted set of Kronecker coefficients.
Joint work with Anirban Chowdhury, David Gosset, Vojtech Havlicek, and Guanyu Zhu
Speaker: John Preskill, QSA, Caltech, 4:20-5:00, Discussion 5:00-5:10
Title: Finding local minima and certifying quantum states
I’ll describe two recent results from our group at Caltech. (1) Finding local minima of the energy in quantum systems is quantumly easy and classically hard. (2) Single-qubit measurements suffice for efficiently certifying that a state in the lab is close to a desired target state.
References:
Local minima in quantum systems, Chi-Fang Chen, Hsin-Yuan Huang, John Preskill, Leo Zhou, arXiv:2309.16596.
Certifying almost all quantum states with few single-qubit measurements, Hsin-Yuan Huang, John Preskill, Mehdi Soleimanifar, arXiv:2404.07281.
Speaker: Eleanor Crane, C2QA, MIT, 5:10-5:25, Discussion 5:25-5:30
Title: Qubit-Boson Quantum Computation for Simulating Fermions, Bosons & Gauge Fields
Abstract: Finding a scalable and universal framework for quantum simulation of strongly correlated fermions and bosons is important in fields ranging from material science to high-energy physics. While digital qubit-only quantum computers in principle offer such universality, the overhead encountered in mapping bosons to qubits renders this endeavour extremely challenging to implement in practice. Here, we develop an approach to simulating bosonic matter, fermionic matter, and Abelian gauge fields in (2+1)D using hybrid digital qubit-boson operations, which avoids this overhead altogether. We show how our compilation strategies can be used to study dynamics as well as ground states and develop measurement techniques of non-local observables such as string-order correlators and the superfluid stiffness. To benchmark our approach, we numerically simulate experiments for a Z2 and a U(1) model including the main sources of hardware noise for superconducting qubits coupled to high-Q cavities, showing that near-term devices can observe phase transitions in these models, also through the use of error mitigation based on Gauss' law. Finally, we perform a gate complexity analysis, finding that a qubit-only implementation requires vastly higher gate counts than our proposal. While our work focuses on superconducting qubit architectures, our tools can be straightforwardly generalized to other platforms hosting controllable bosonic modes such as trapped ions and neutral atoms in tweezer arrays. Our work shows the key advantages of hybrid qubit-boson hardware to simulating bosonic models such as lattice gauge theories and presents a clear manual to compile fermions, bosons and gauge fields onto this hardware.
End of Day 5:30
Tuesday, May 21st
Speaker: Sasha Boltasseva, QSC, Purdue, 9:00-9:40, Discussion 9:40-9:50
Title: Advancing Nano- and Quantum Photonics with Machine Learning
Abstract: Discovering unconventional optical designs via machine-learning promises to advance on-chip circuitry, imaging, sensing, energy, and quantum information technology. In this talk, we discuss photonic design approaches and emerging material platforms for showcasing machine-learning-assisted topology optimization for optical metasurface designs with applications in thermophotovoltaics, reflective optics, quantum photonic circuitry and lightsail technology. We demonstrate the effectiveness of autoencoders for compressing the vast design space of metasurfaces into a smaller search space. By employing global optimization via adjoint methods or quantum annealing, one can find the optimal metasurface designs within the smaller space constructed by the autoencoder. The techniques employed in this work extend well beyond the metasurface optimization space and into many inverse design problems for engineering and physics. We also apply machine learning approaches to advance quantum measurements and superresolution imaging.
Speaker: Tom Iadecola, SQMS, Iowa State, 9:50-10:30, Discussion 10:30-10:40
Title: Robust preparation of ground state phases under noisy imaginary time evolution
Abstract: Nonunitary state preparation protocols such as imaginary time evolution (ITE) offer substantial advantages relative to unitary ones, including the ability to prepare certain long-range correlated states more efficiently. Here, we ask whether such protocols can also be more robust to noise from coupling to the environment. We consider a nonunitary ITE “circuit” subjected to a variety of noise models and ask whether the resulting steady state remains in the same phase as the target state of the ITE at finite noise strength. Taking the one-dimensional quantum Ising model as a concrete example, we find that the ground state order and associated phase transition persist in the presence of noise, provided the noise does not explicitly break the symmetry that protects the phase transition. That is, the noise must possess the protecting symmetry as an “average” or “weak” symmetry. Our analysis is facilitated by a mapping to an effective Hamiltonian picture in a doubled Hilbert space. We discuss how noise tailoring strategies including Pauli twirling can be utilized to enforce the desired average symmetry, as well as possible implications for calculations on noisy quantum computers.
Break 10:40-11:00 (light refreshments)
Speaker: Fred Chong, QNEXT, U Chicago & Infleqtion, 11:00-11:40, Discussion 11:40-11:50
Seymour Goodman Professor, University of Chicago
Chief Scientist for Quantum Software, Infleqtion
Title: Physics-Aware Co-Design and Optimization of Quantum and Hybrid Applications and Protocols
Abstract: Quantum software can be a force multiplier that can significantly shorten the timeline for utility-scale results from quantum hardware. In particular, several key research directions will help realize practical quantum advantage. Physics-aware, cross-layer optimizations will continue to yield important efficiencies to allow applications to make the most of quantum resources. Software-directed error mitigation, in particular, will be key to increasing gate depths and maintaining acceptable output fidelity. Pulse-level optimizations and specialized native gates will
also be key enablers. Additionally, applications will be hybrid computations involving high-performance classical resources as well as quantum hardware serving as special-purpose accelerators. Effectively partitioning computations between these classical and quantum resources will be necessary to support realistic applications. Finally, deep compiler optimization and classical simulation of Clifford and near-Clifford circuits can also be important classical investments towards more efficient quantum computations.
Lunch 11:50-1:40 (boxed lunch)
Speaker: Antonios Alvertis, SQMS, Lawrence Berkeley NL, 1:40-1:55, Discussion 1:55-2:00
Title: Classical benchmarks for variational quantum eigensolver simulations of strongly correlated
Abstract: Simulating the Hubbard model is of great interest to a wide range of applications within condensed matter physics and beyond, however its solution on classical computers remains challenging in dimensions larger than one. The relative simplicity of this model, embodied by the sparseness of the Hamiltonian matrix, allows for its efficient implementation on quantum computers, and for its approximate solution using variational algorithms such as the variational quantum eigensolver. However, there remain several unknowns, such as the accuracy of these algorithms with increasing system size and interaction strength, the choice of variational ansatz, and the degree of spatial inhomogeneity in the model. Here we present a rigorous classical benchmarking study, demonstrating the potential impact of these factors on the accuracy of the variational solution of the Hubbard model on quantum hardware. We find that even when using the most accurate wavefunction ans\"{a}tze known for the Hubbard model, the error in its ground state energy plateaus for larger lattices, while stronger electronic correlations magnify this issue. Concurrently, spatially inhomogeneous parameters do not reduce the accuracy of the computed ground state energies. Our study highlights the capabilities and limitations of current approaches for solving the Hubbard model on quantum hardware, and we discuss potential future avenues of research.
Speaker: Mark Saffman, UW-Madison and Infleqtion, Inc., 2:00-2:15, Discussion 2:15-2:20
Title: Architecture for fast implementation of qLDPC codes with optimized Rydberg gates
Abstract: We propose an implementation of bivariate bicycle codes (Nature 627, 778 (2024)) based on long-range Rydberg gates between stationary neutral atom qubits. An optimized layout of data and ancilla qubits reduces the maximum Euclidean communication distance needed for non-local parity check operators. An optimized Rydberg gate pulse design enables CZ entangling operations with fidelity F > 0.999 at a distance greater than 12 μm. The combination of optimized layout and gate design leads to the potential for significant reduction of the quantum error correction cycle time for a [[144, 12, 12]] code compared to previous neutral atom designs.
Speaker: Yigit Subasi, QSC, Los Alamos NL, 2:20-2:35, Discussion 2:35-2:40
Title: Optimal Coherent Quantum Phase Estimation via Tapering
Abstract: We study the coherent version of the phase estimation problem, where given an arbitrary input state and black-box access to unitaries U and controlled-U, the goal is to estimate the phases of U in superposition. We propose an improved version of the standard algorithm that utilizes tapering/window functions. Our algorithm achieves the optimal query complexity (total number of calls to U and controlled-U) without requiring the use of a computationally expensive quantum sorting network for median computation, which the standard algorithm uses to boost the success probability arbitrarily close to one. We also show that the tapering functions that we use are optimal by formulating optimization problems with different optimization criteria. Finally, we also propose an efficient algorithm that prepares the quantum state corresponding to the optimal tapering function.
Speaker: Manas Sajjan, QSC, Purdue University, 2:40-2:55, Discussion 2:55-3:00
TBD
Break 3:00-3:30 (light refreshments)
Speaker: Yanzhu Chen, C2QA, Virginia Tech, 3:30-4:10, Discussion 4:10-4:20
Title: Adaptive quantum algorithms
Abstract: Many applications of quantum computing can be formulated as the problem of finding the ground state for a given Hamiltonian. Variational quantum algorithms provide a promising approach to such problems, where the performance relies on the quality of the variational ansatz. The Adaptive Derivative-Assembled Problem-Tailored (ADAPT) strategy has been developed to select the most relevant operators in the ansatz. The ansatz and the optimized parameters determine what quantum circuit will be used to prepare the ground state. In this talk I will briefly review the application of the ADAPT strategy in finding or approximating the ground state for molecules and classical combinatorial problems, followed by a discussion of some recent developments. Specifically, for combinatorial problems, the ADAPT strategy can be employed together with a classically inspired initial state, providing further improvement in the performance. Moreover, using the ADAPT strategy, we can construct a quantum circuit to approach the ground state without the variational procedure, circumventing the difficulty in classical optimization and potentially saving measurement resources.
Speaker: Peter Love, QSA, Tufts U, 4:20-5:00, Discussion 5:00-5:10
Title: Quantum simulation of quantum field theory on the light-front
Abstract: Quantum simulation is a leading future application of quantum computation. Quantum field theory poses novel challenges because of the need to regulate and renormalize the theories in order to extract physically meaningful results. Quantum simulation algorithms also require a Hamiltonian formulation of the theory. Dirac showed that relativistic theories admit three Hamiltonian formulations. The equal time formulation is commonly used for quantum field theory, whereas the front form offers several advantages for the computation of properties of composite particles. In this talk I will review work on quantum simulation of quantum field theory in the light front formulation, including questions of renormalization, simulation of scattering processes and quantum resources on the light front.
Break until dinner at Hotel Parq Central 5:00-6:00
Banquet Dinner – 7:00-8:00 (plated dinner of choice)
Dinner Speaker (Hotel Parq Central): Hengyun (Harry) Zhou, Harvard University and QuEra Computing, Inc., May 21st, 7:00-8:00
Title: Testing Quantum Algorithms using Neutral Atom Quantum Computers
Abstract: In this talk, I will give an overview of neutral atom quantum computers, an emerging platform for realizing and testing quantum algorithms with both physical and logical qubits. The talk will start by introducing the basic building blocks of neutral atom quantum computer operations and describing some differences from other platforms. Central to our approach is the concept of co-design, involving a combination of algorithms and hardware-efficient implementations as well as appropriate quantum error correcting codes. I will specifically highlight some of the key characteristics that make this system particularly well-suited for error-corrected computations, including recent experimental results on complex logical algorithms with up to 48 logical qubits, and exciting opportunities to realize and test large scale algorithms using fault-tolerant quantum computers.
Wednesday, May 22nd
Speaker: Lukasz Cincio, QSC, Los Alamos NL, 9:00-9:40, Discussion 9:40-9:50
Title: Solving Materials Science Problems with Quantum Computers
Abstract: It has been twenty years of quantum computing research, and it is still not clear whether quantum computers can solve important problems in materials science. There are many obstacles that current quantum algorithms are facing. In particular, they only apply to a limited number of simulation problems and they assume properties of models that are not met in real-life scenarios. Furthermore, their complexities have been derived in the limit of infinite system size, which can be substantially different in practical situations. As a consequence, current requirements to achieve quantum advantage for problems in material science (where a quantum computer surpasses its classical counterpart) are not realistic. I will be discussing practical methods and techniques for solving materials science problems by current noisy and future fault-tolerant quantum computers. Those problems include classifying quantum phases of matter and real-time dynamics. I will be focusing on problems that appear to be best candidates for achieving quantum advantage, which naturally fit on existing and future quantum-computer hardware but are simultaneously intractable for classical computers.
Speaker: Henry Lamm, SQMS, Fermilab, 9:50-10:30, Discussion 10:30-10:40
TBD
Break 10:40-11:00 (light refreshments)
Speaker: Bryan Clark, QNEXT, UIUC, 11:00-11:40, Discussion 11:40-11:50
TBD
Lunch 11:50-1:40 (buffet style Mediterranean)
Speaker: Oluwadara A. Ogunyoka, SQMS, Fermilab, 1:40-1:55, Discussion 1:55-2:00
Title: Qutrit circuits and algebraic relations: A pathway to efficient spin-1 Hamiltonian simulation
Abstract: Quantum information processing has witnessed significant advancements through the application of qubit- based techniques within universal gate sets. Recently, exploration beyond the qubit paradigm to d-dimensional quantum units or qudits has opened new avenues for improving computational efficiency. This talk delves into the qudit-based approach, particularly addressing the challenges presented in the high-fidelity implementation of qudit-based circuits due to increased complexity. As an innovative approach towards enhancing qudit circuit fidelity, we explore algebraic relations, such as the Yang-Baxter-like turnover equation, which may enable circuit compression and optimization. The talk introduces the turnover relation for the three-qutrit time propagator and its potential use in reducing circuit depth. We further investigate whether this relation can be generalized for higher-dimensional quantum circuits, including a focused study on the one-dimensional spin-1 Heisenberg model. This talk outlines both rigorous and numerically efficient approaches to potentially achieve this generalization, providing a foundation for further explorations in the field of qudit-based quantum computing.
Speaker: Andrew Baczewski, QSA, Sandia NL, 2:00-2:15, Discussion 2:15-2:20
Title: Quantum computation of stopping power for inertial fusion target design
Abstract: Stopping power is the rate at which a material absorbs the kinetic energy of a charged particle passing through it -- one of many properties needed over a wide range of thermodynamic conditions in modeling inertial fusion implosions. First-principles stopping calculations are classically challenging because they involve the dynamics of large electronic systems far from equilibrium, with accuracies that are particularly difficult to constrain and assess in the warm-dense conditions preceding ignition. Here, we describe a protocol for using a fault-tolerant quantum computer to calculate stopping power from a first-quantized representation of the electrons and projectile. Our approach builds upon the electronic structure block encodings of Su et al. [PRX Quantum 2, 040332 2021], adapting and optimizing those algorithms to estimate observables of interest from the non-Born-Oppenheimer dynamics of multiple particle species at finite temperature. Ultimately, we report logical qubit requirements and leading-order Toffoli costs for computing the stopping power of various projectile/target combinations relevant to interpreting and designing inertial fusion experiments. We estimate that scientifically interesting and classically intractable stopping power calculations can be quantum simulated with roughly the same number of logical qubits and about one hundred times more Toffoli gates than is required for state-of-the-art quantum simulations of industrially relevant molecules such as FeMoco or P450.
Speaker: Kevin Smith, C2QA, Yale, 2:20-2:35, Discussion 2:35-2:40
Title: Speeding up state preparation with adaptive quantum circuits
Abstract: Matrix product states (MPS) comprise a broad class of physically interesting entangled states highly relevant to condensed matter physics, quantum chemistry, and quantum machine learning. While it is well known that any matrix product state can be exactly prepared using a linear-depth circuit, decoherence limits current NISQ-era processors to fairly shallow-depth circuits, inhibiting the preparation of MPS larger than a few sites. In this talk, I will show that so-called adaptive quantum circuits, which augment unitary circuits with non-unitary resources such as mid-circuit measurement and feedforward operations, can be used to exactly prepare a diverse family of MPS in constant depth — an impossible feat with unitary resources alone. I will discuss the preparation of the AKLT state as a particular instance of our general protocol, and will furthermore discuss its extension to a broad family of MPS that includes symmetry-protected topological and symmetry broken states, MPS with finite abelian, non-abelian, and continuous symmetries, and resources states for measurement-based quantum computation.
Speaker: Andrew J. Landahl, QSA, Sandia NL and University of New Mexico, 2:40-2:55, Discussion 2:55-3:00
Title: Hunting for sexaquark dark matter with a fault-tolerant quantum computer
Abstract: I will discuss a potential exotic hexaquark hadron in the Standard Model with the racy name “sexaquark,” highlighting how if its mass is in the right range, it could be the long-awaited answer to what dark matter is. I will then discuss why it is so hard to calculate its mass with conventional techniques like lattice QCD. This leads into my presentation of an approach my colleagues and I developed that is amenable to fault-tolerant quantum computers, namely an algorithm that can compute many properties of the structure of relativistic bound states of hadrons including form factors, parton distribution functions, and generalized parton distributions. The approach uses Dirac’s light-front formulation of quantum field theory, in which one uses a coordinate system moving at the speed of light. I will conclude by describing our algorithm in detail, along with concrete estimates for how many resources such a quantum computer would need to run the algorithm. With any luck, some day a quantum computer running our algorithm will perform this ab initio calculation of the sexaquark’s mass, charting the way for searches for this particle that has yet to be ruled out experimentally or observationally.
Break: 3:00-3:30
Speaker: Kaitlin Smith, QNEXT, Northwestern University, 3:30-4:10, Discussion 4:10-4:20
Title: Exploring the Frontier of Chiplet-based Quantum Systems
Abstract: Quantum systems based on modular architectures provide a viable path for scaling. Particularly, systems based on locally-connected chiplets have potential to drastically increase the number of physical qubits available in the near term. In this talk, we will first review the benefits of chiplet-based quantum systems. Next, we will discuss challenges and opportunities associated with applying modular systems toward practical quantum computing.
Speaker: Akimasa Miyake, QSA, UNM, 4:20-5:00, Discussion 5:00-5:10
Title: Group-theoretic error mitigation enabled by classical shadows and symmetries
Abstract: Together with rapid developments of quantum error correction and mitigation, it remains important to explore the reduction of space-time overhead as well as understand the tradeoff of overhead to negate errors in running quantum algorithms and simulation on near-term quantum devices. Because most of system Hamiltonians of interest have a symmetry such as global U(1) symmetry, we propose symmetry-adjusted classical shadows, where group theory enables us to adjust classical-shadow tomography according to how symmetries are corrupted by errors. We compare ours with other symmetry-based mitigation methods, and find that symmetry-adjusted classical shadows are a low-overhead strategy to improve precision in state of the art experiments with noise strength typically between 1% and 0.1%. The talk is based on a joint work with Andrew Zhao that was posted as arXiv:2310.03071 and appears in npj Quantum Information.